Validation
The following is a compilation of validation studies found in the literature using FLOWUnsteady.
Wing
Sources: E. J. Alvarez, 2022,[1] and E. J. Alvarez and A. Ning, 2023[2]
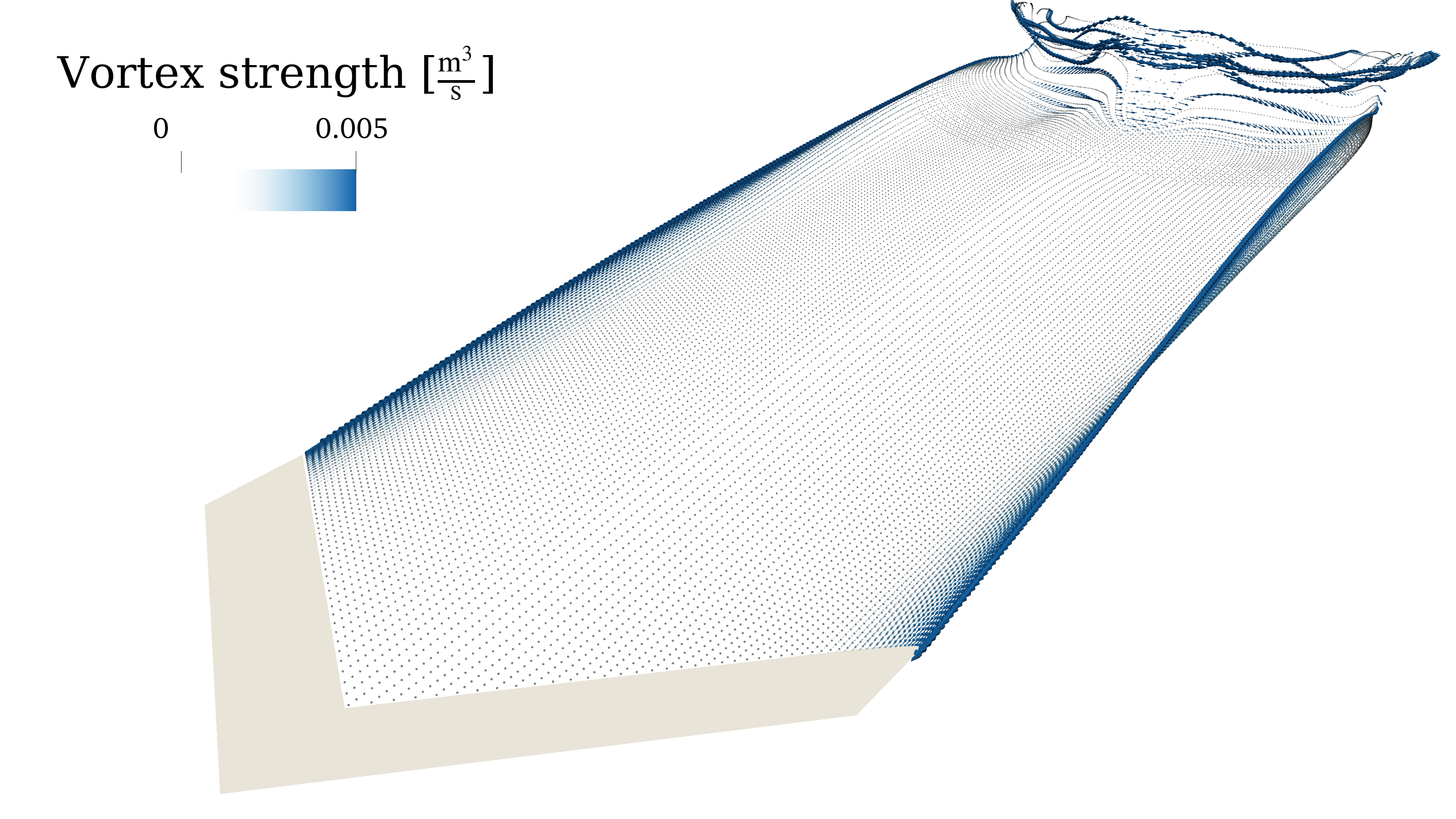
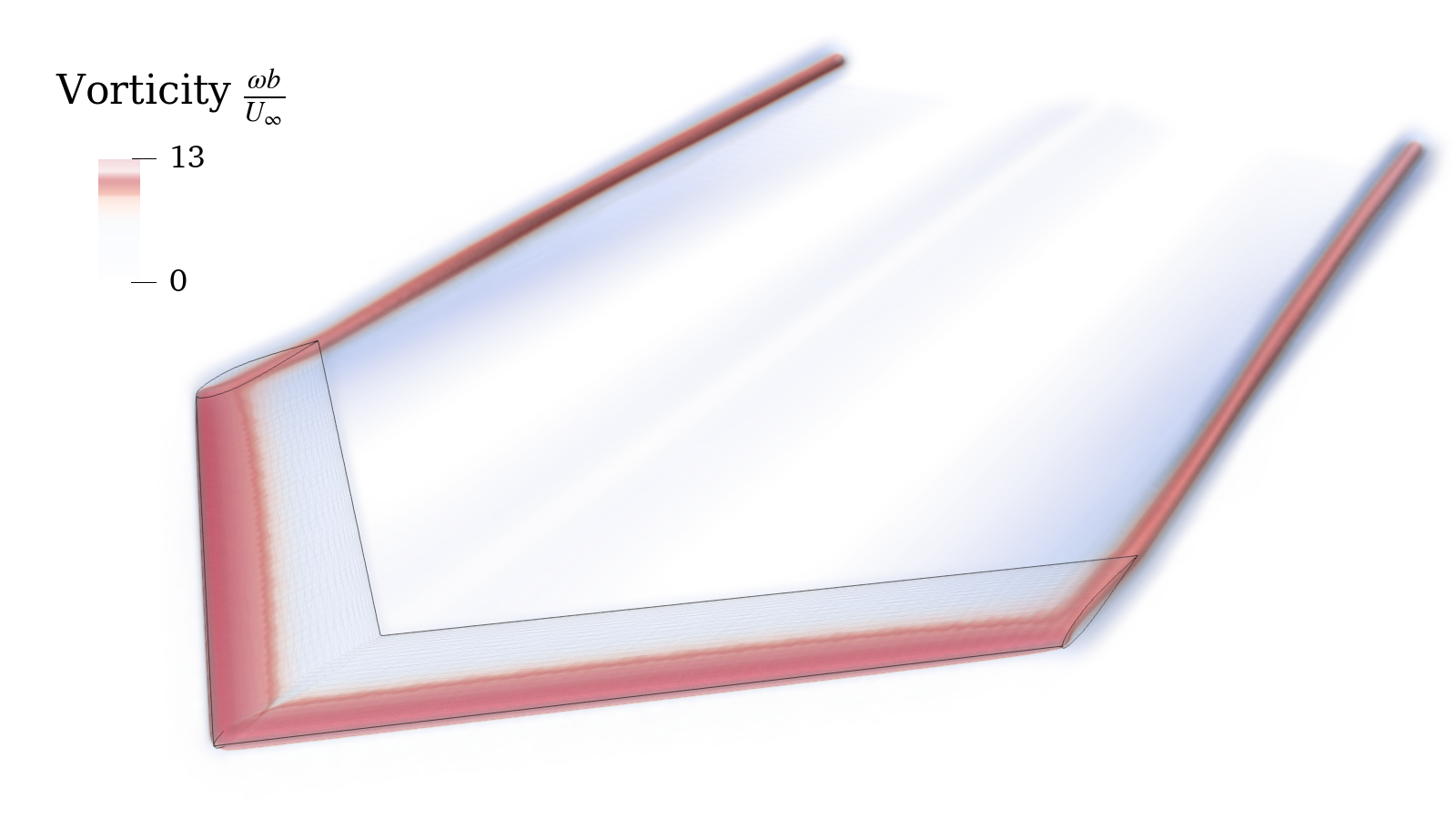
Case: $45^\circ$ swept-back wing at an angle of attack of $4.2^\circ$, aspect ratio of 5.0, RAE 101 airfoil section with 12% thickness, no dihedral, twist, nor taper. Freestream velocity of $49.7,\mathrm{m/s}$, corresponding to a chord-based Reynolds number of $1.7 \times 10^6$. The high sweep of the wing causes non-negligible spanwise flow. The wing loads reported by Weber and Brebner (experimental) were integrated from pressure-tap measurements, hence the drag reported in this section includes induced and form drag while excluding skin friction drag.
Results: (Excerpt from E. J. Alvarez and A. Ning, 2022[2]) "Fig. 9 shows the loading distribution and integrated lift and drag across AOA predicted with the actuator surface model (ASM), compared to the experimental measurements. The loading distribution shows satisfactory agreement with the experiment, validating that both the circulation solver and the force calculation... are accurate for predicting not only lift but also drag distribution across the span. The integrated lift and drag (Fig. 9, bottom) show excellent agreement with the experiment from $0^\circ$ to $10.5^\circ$. We expect this to be the case only for mild AOAs before approaching stall conditions since our ASM does not capture the mechanisms of flow separation. Thus, through this swept-wing case, we gain confidence that our ASM yields accurate predictions in conditions with spanwise flow up to a moderate AOA."
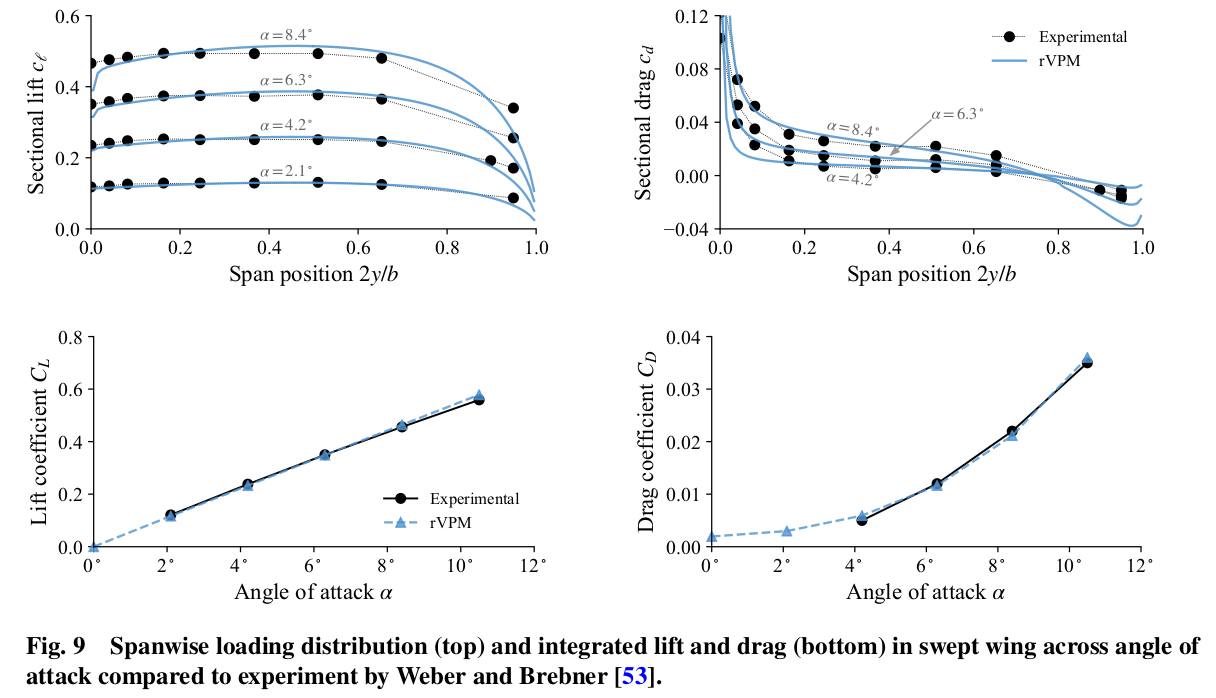
Rotor
Hover Case: Aero
Source: E. J. Alvarez, 2022[1], and E. J. Alvarez and A. Ning, 2022[6]
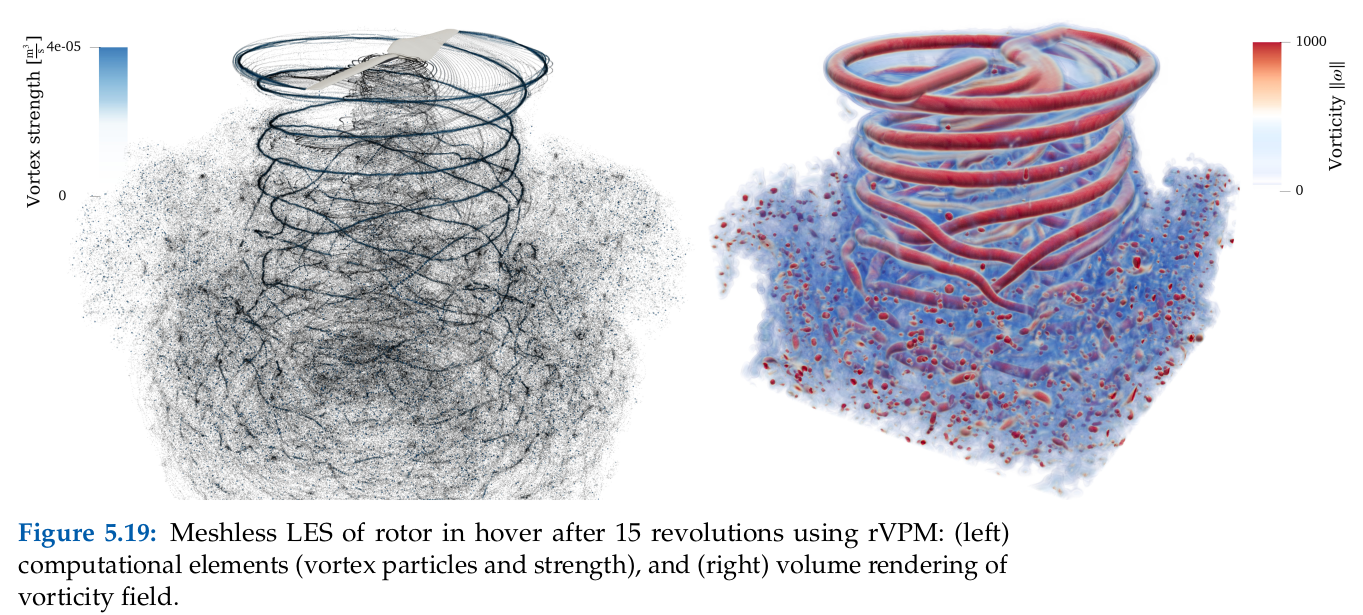
Case: DJI 9443 rotor in hover at 5400 RPM. This is a two-bladed rotor with a diameter of 9.4 inches. Validation case at Mach number of 0.2, $\mathrm{Re}_c = 6 \times 10^4$, and $\mathrm{Re}_{0.7D} = 7 \times 10^5$. Thrust and blade loading are compared to experiment and mesh-based CFD. Computational time is benchmarked against conventional mesh-based CFD.
Results: (Excerpt from E. J. Alvarez and A. Ning, 2020[6]) "...the VPM simulation shows excellent agreement with the experiment, predicting a mean $C_T$ value within 2% of the experimental mean value... Our meshless LES appears to be two orders of magnitude faster than a mesh-based LES with similar fidelity, while being one order of magnitude faster than a low-fidelity URANS simulation and three orders of magnitude than high-fidelity DES."
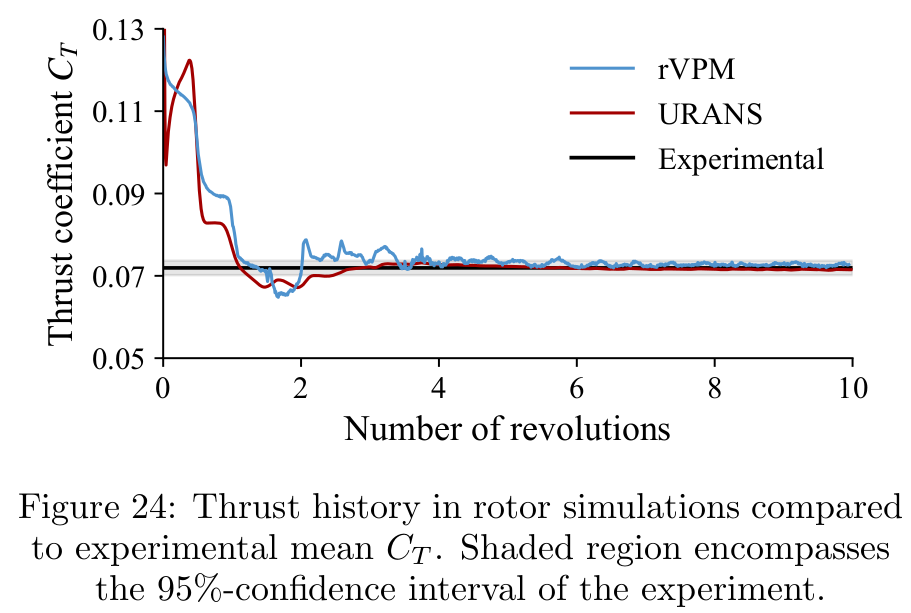
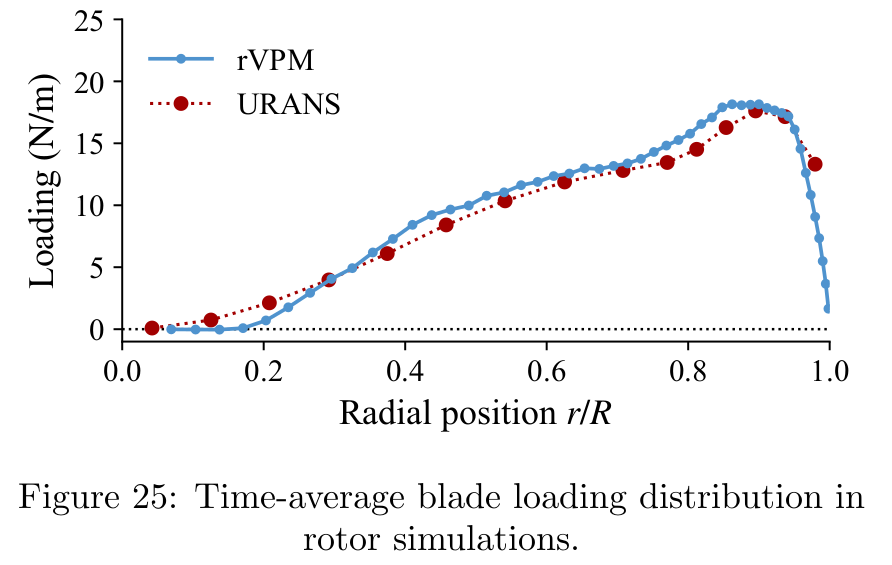
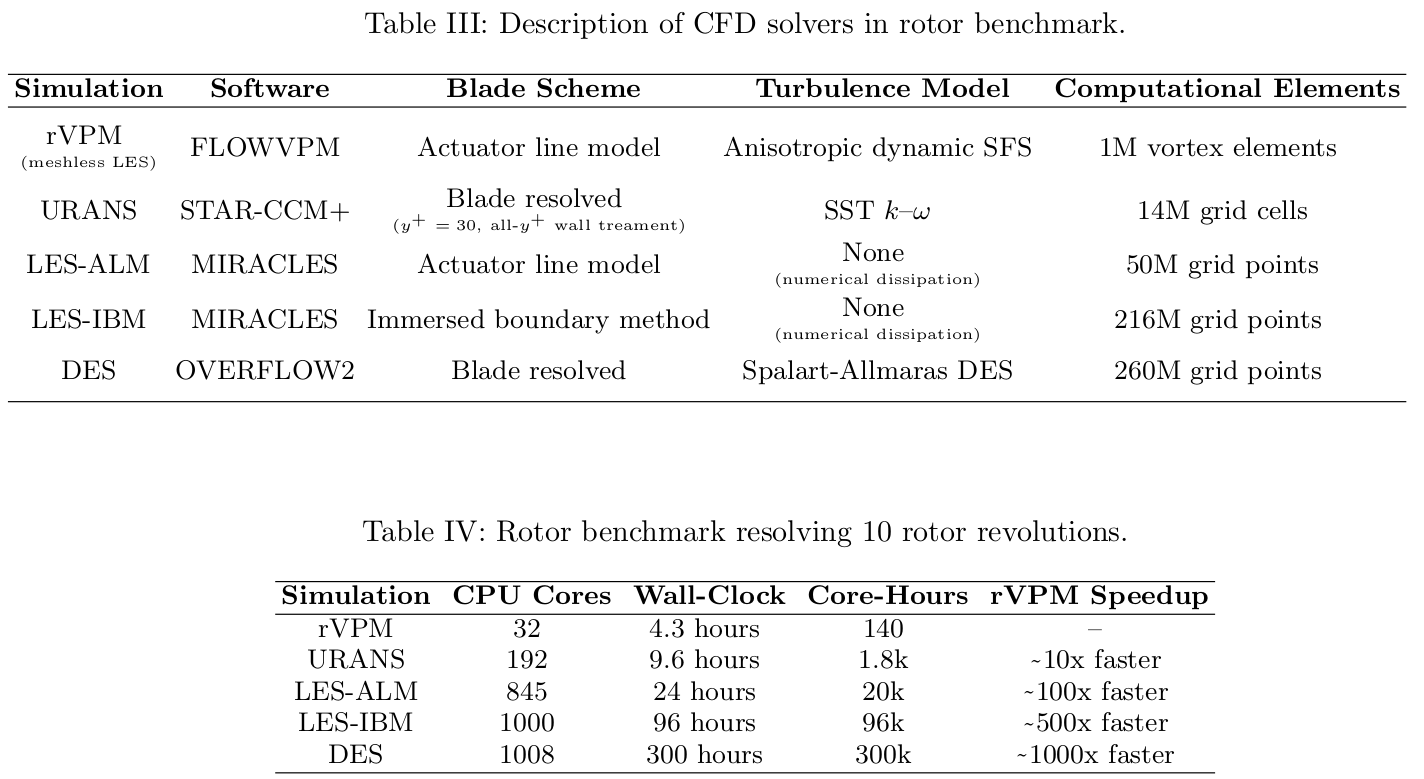
Hover Case: Acoustics
Source: E. J. Alvarez, A. Schenk, T. Critchfield, and A. Ning, 2020[7]
Case: Same DJI 9443 case in hover. FLOWUnsteady predictions (VPM) are compared against experiment and URANS (STAR-CCM+) and DES (OVERFLOW2).
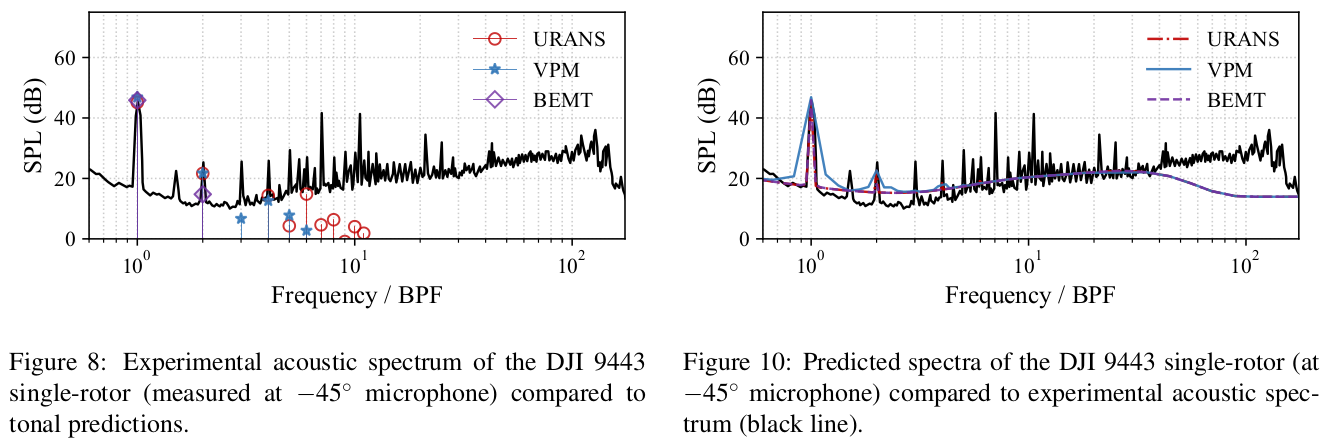
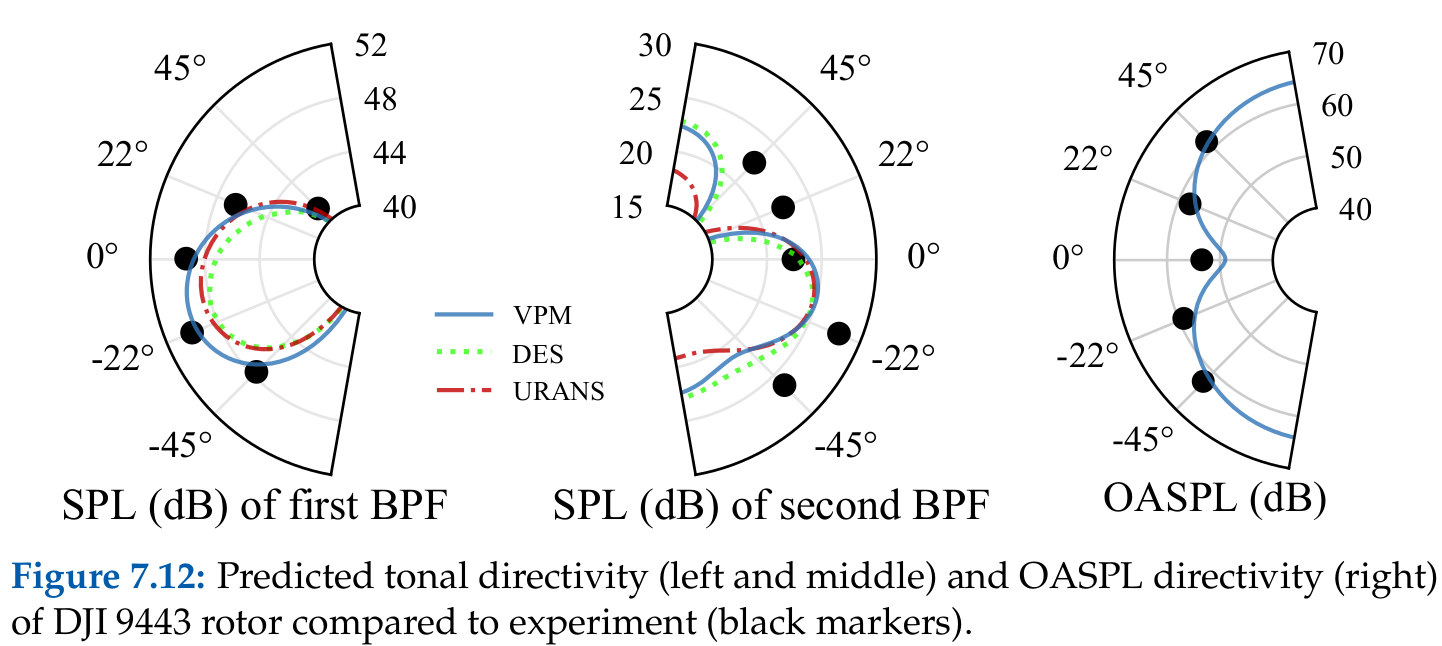
NOTE: The broadband results in E. J. Alvarez, A. Schenk, T. Critchfield, and A. Ning, 2020[7] contained a bug that ended up evaluating the microphone array in the wrong plane, which led to a large discrepancy in A-weighted OASPL. For updated results, see the Rotor in Hover tutorial.
Forward Flight Case
Source: R. M. Erhard and J. J. Alonso, 2022[8]
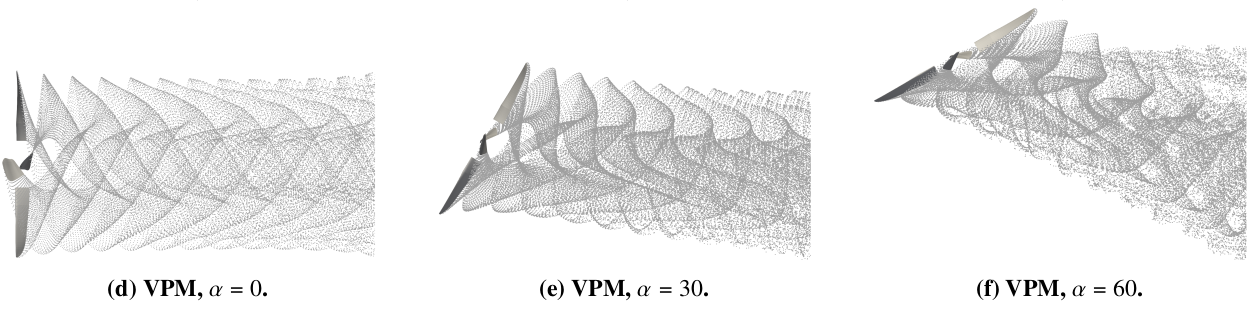
Case: TUD F29 rotor in forward flight at an incidence angle (edgewise flow). This is a four-bladed rotor. Thrust and power predicted by FLOWUnsteady (VPM) was compared to experiment and low-fidelity methods at multiple incidence angles $\alpha$ and advance ratios $J$.
Results: Good agreement between FLOWUnsteady and experiment for incidence angles up to $\alpha=60^\circ$ across advance ratio.
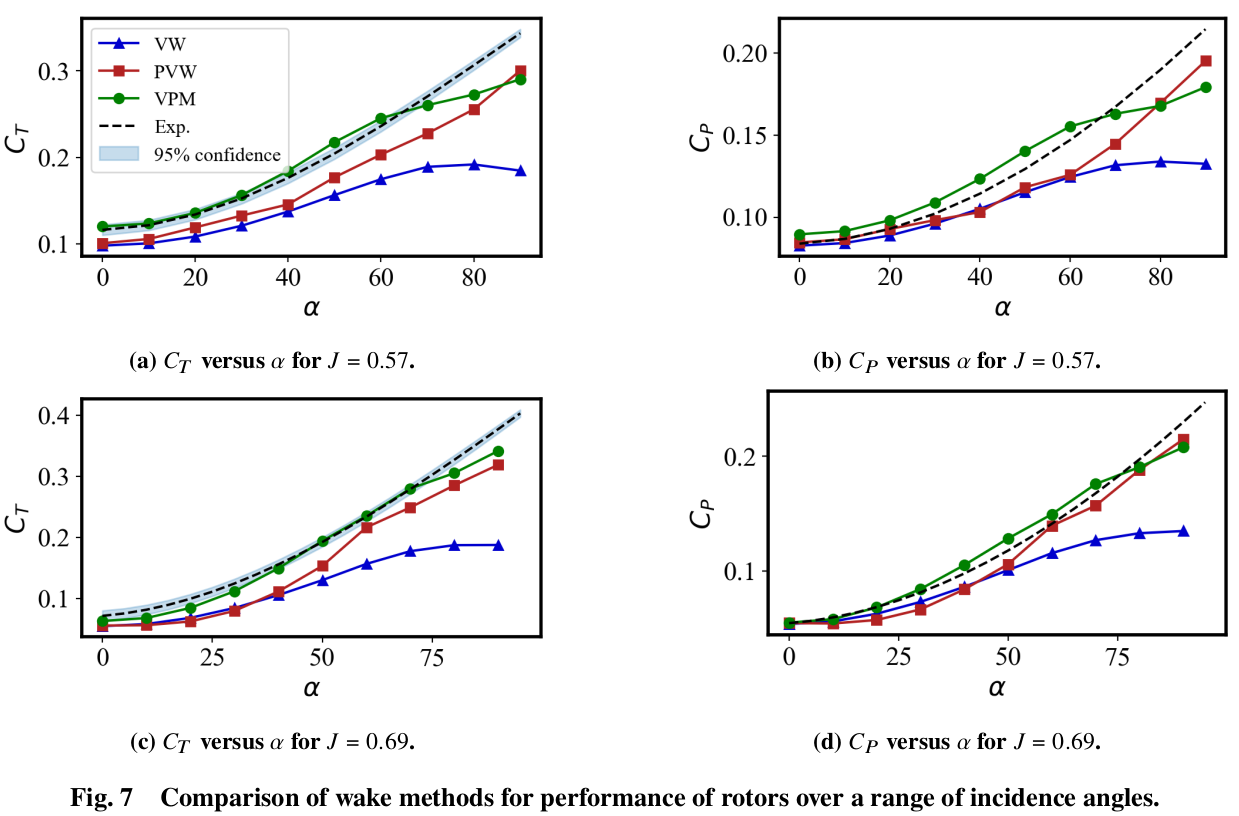
Wind Turbine
Source: J. Mehr, E. J. Alvarez, and A. Ning, 2022[5]
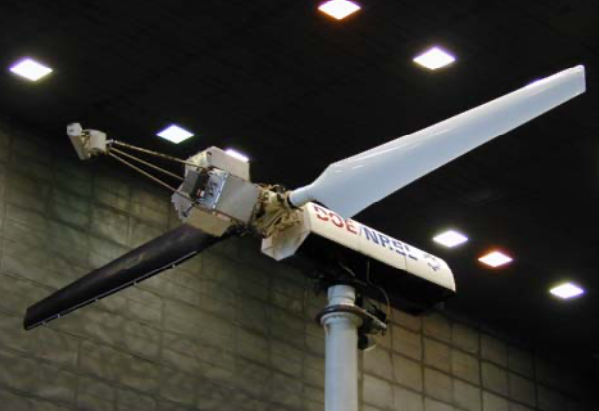
Case: Test series "H" from UAE study at US Department of Energy. Sweep on tip speed ratio $\lambda = \frac{\omega R}{u_\infty}$.
Results: (Excerpt from J. Mehr, E. J. Alvarez, and A. Ning, 2020[5]) "The torque coefficient outputs are also within 10% for tip speed ratios above, and within 25% for tip speed ratios under, $\lambda = 2.0$; also notice that the absolute magnitudes of the torque coefficient are very small at low tip speed ratios, assuaging any concerns about the higher relative errors at those operational states."
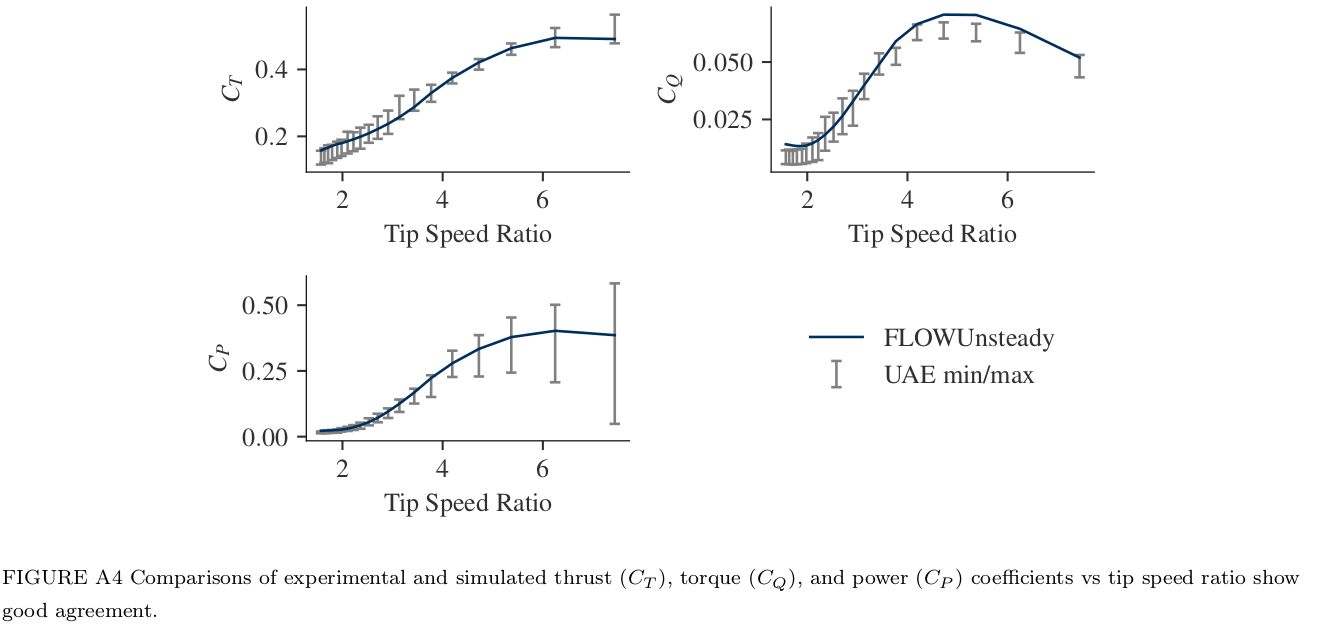
Propeller
APC 10x7 Case
Source: E. J. Alvarez and A. Ning, 2020[3]
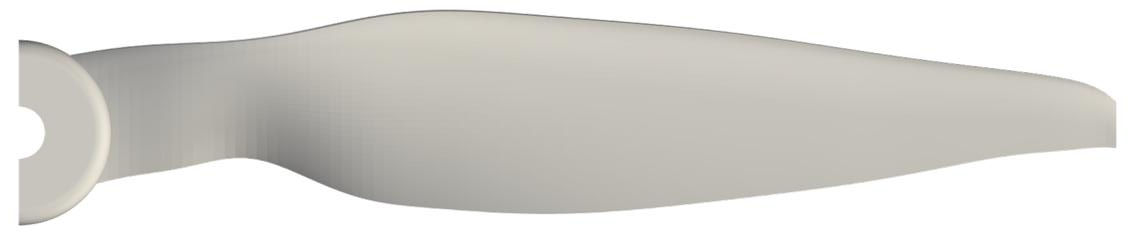
Case: APC 10x7E propeller (2 blades, 10'' diameter, solidity 0.1) at a tip Mach number of 0.36, $\mathrm{Re}_c = 6.2 \times 10^4$, and $\mathrm{Re}_D = 6.5 \times 10^5$. Sweep on advance ratio $J = \frac{u_\infty}{n d}$.
Results: (Excerpt from E. J. Alvarez and A. Ning, 2020[3]) "... it is confirmed that the VPM propeller model is valid... across low and moderately high advance ratios."
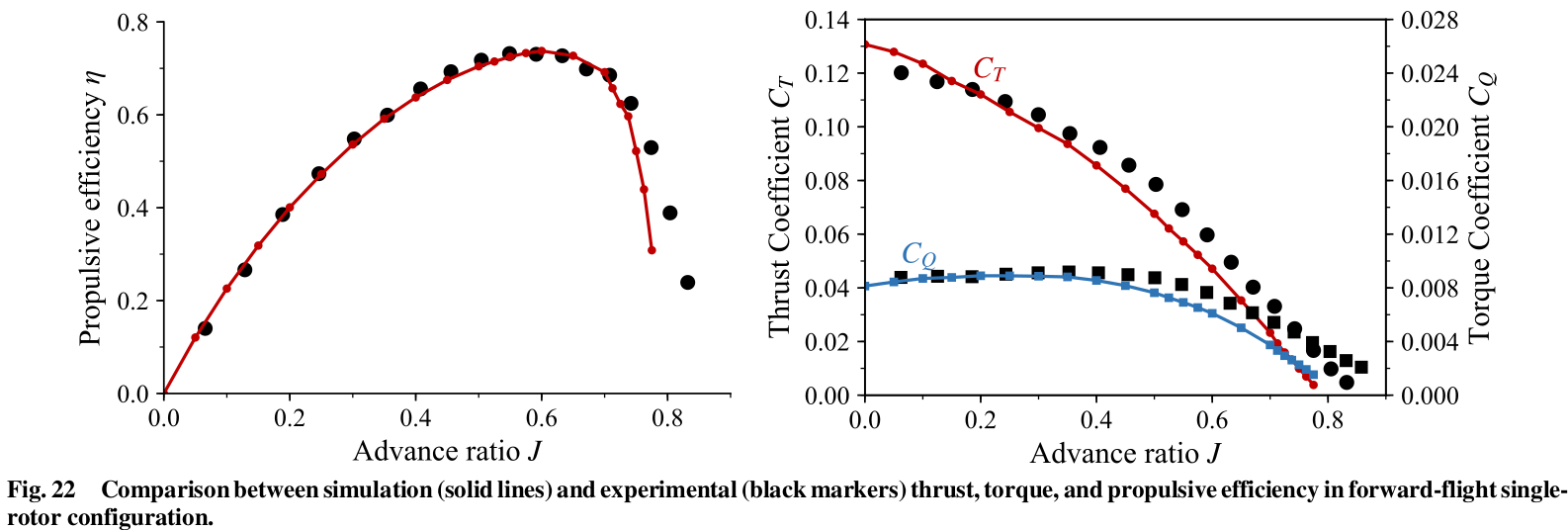
Beaver Case
Source: E. J. Alvarez, 2022[1], and E. J. Alvarez and A. Ning, 2023[2]
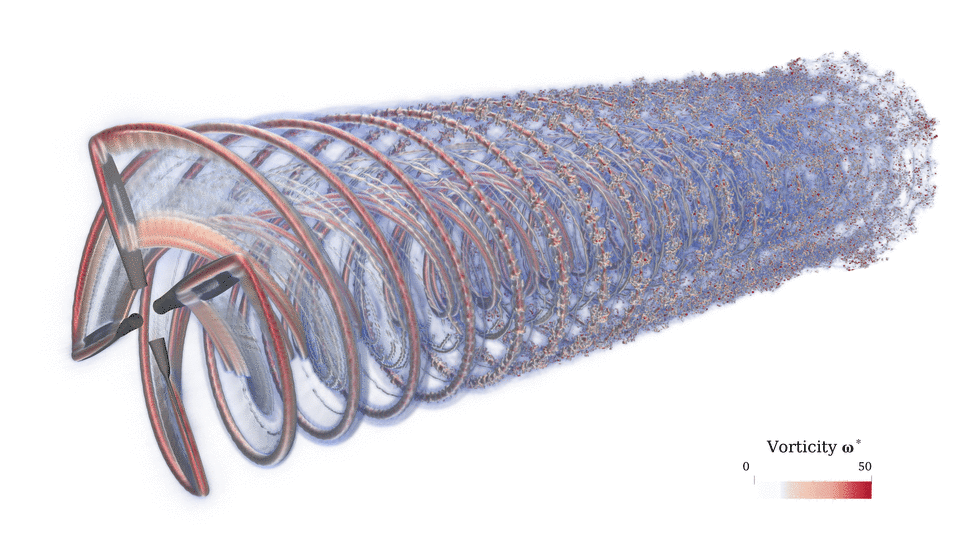
Case: Beaver propeller (4 blades, 0.237 m diameter) at $\mathrm{Re}_{0.7D} = 1.8\times 10^6$. Extensive validation with predicted thrust, torque, efficiency, blade loading, and flow field compared to experimental measurements.
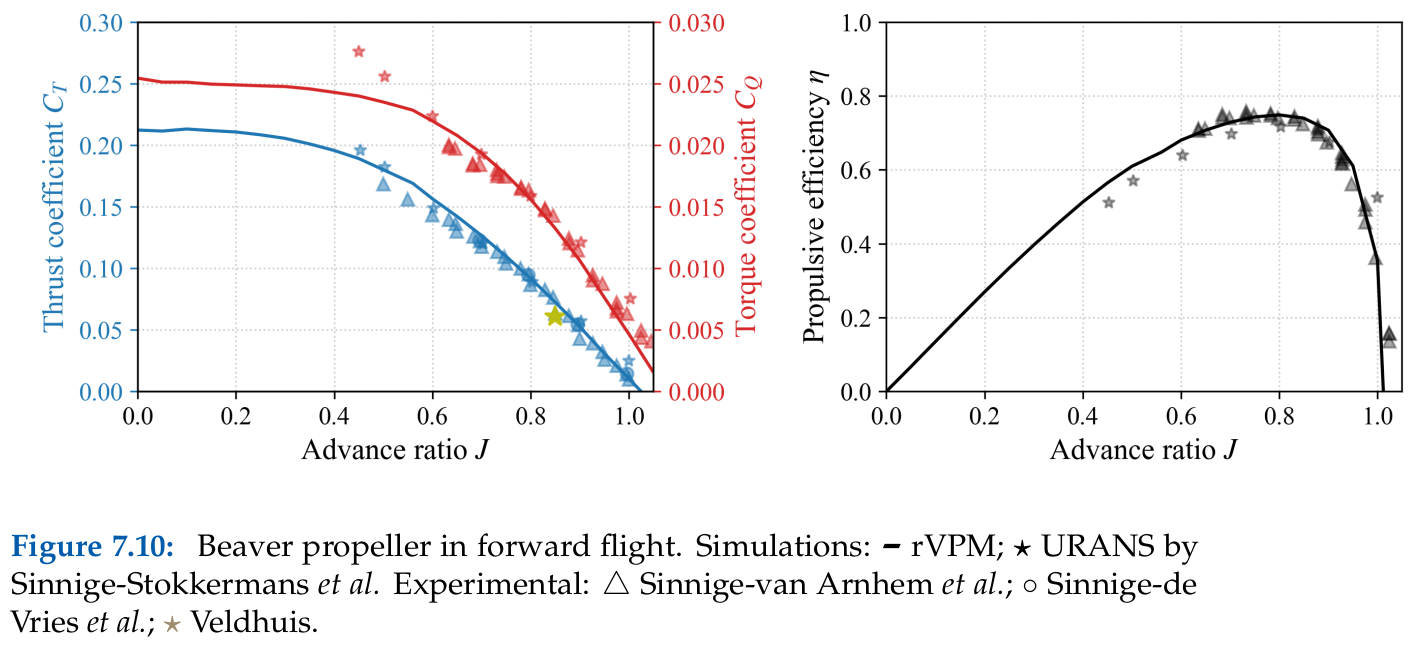
Sweep of incidence angle
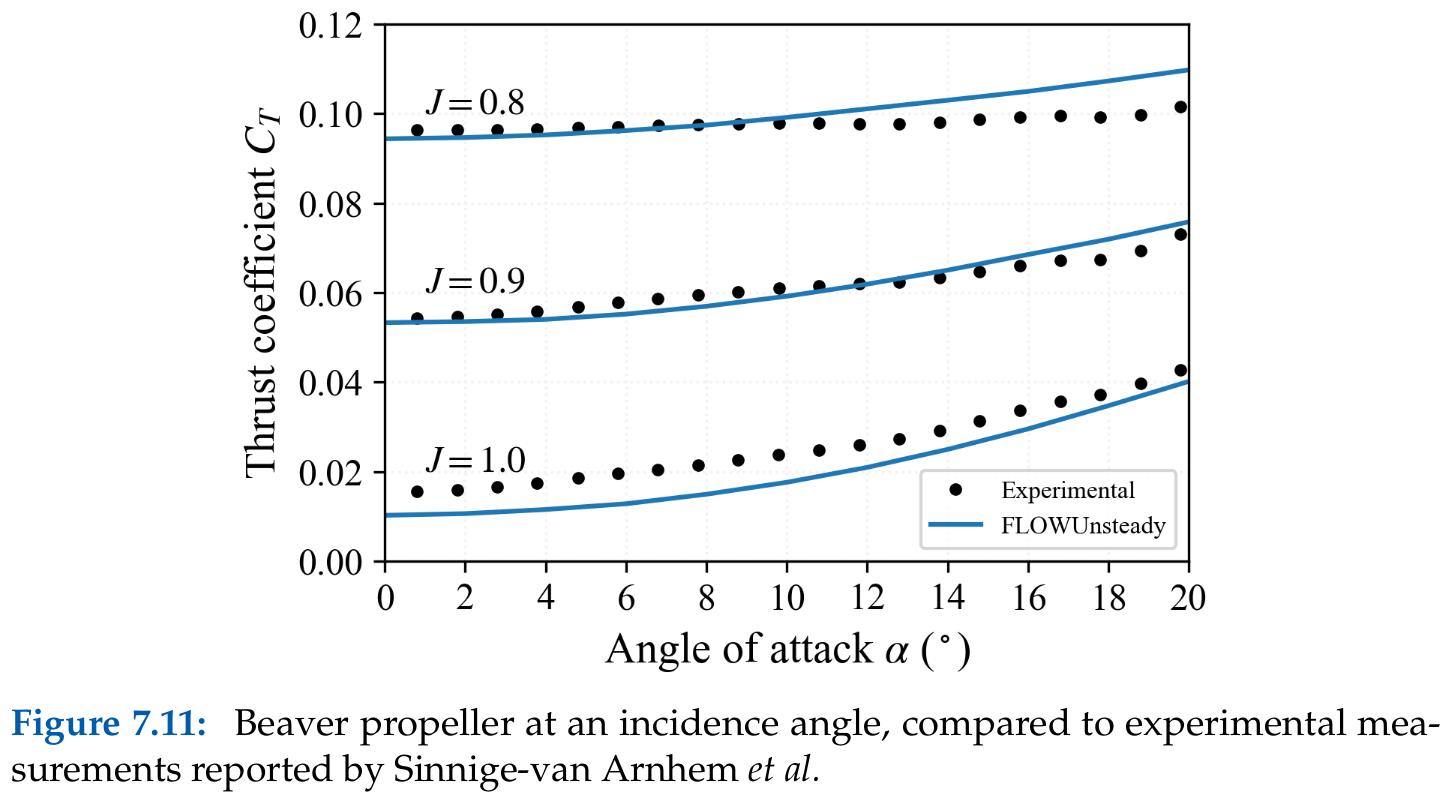
Blade loading
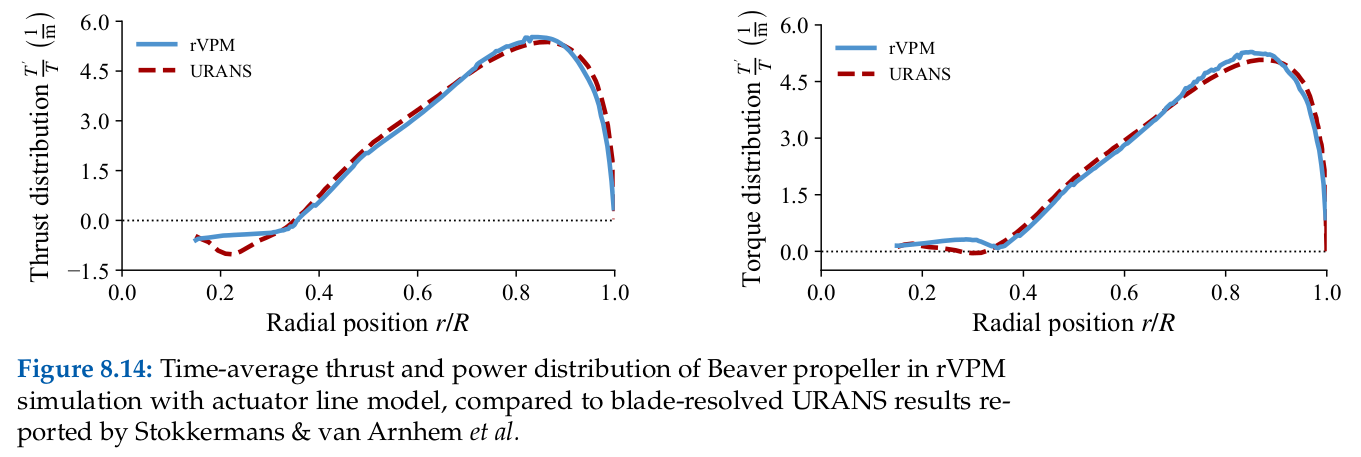
Wake structure
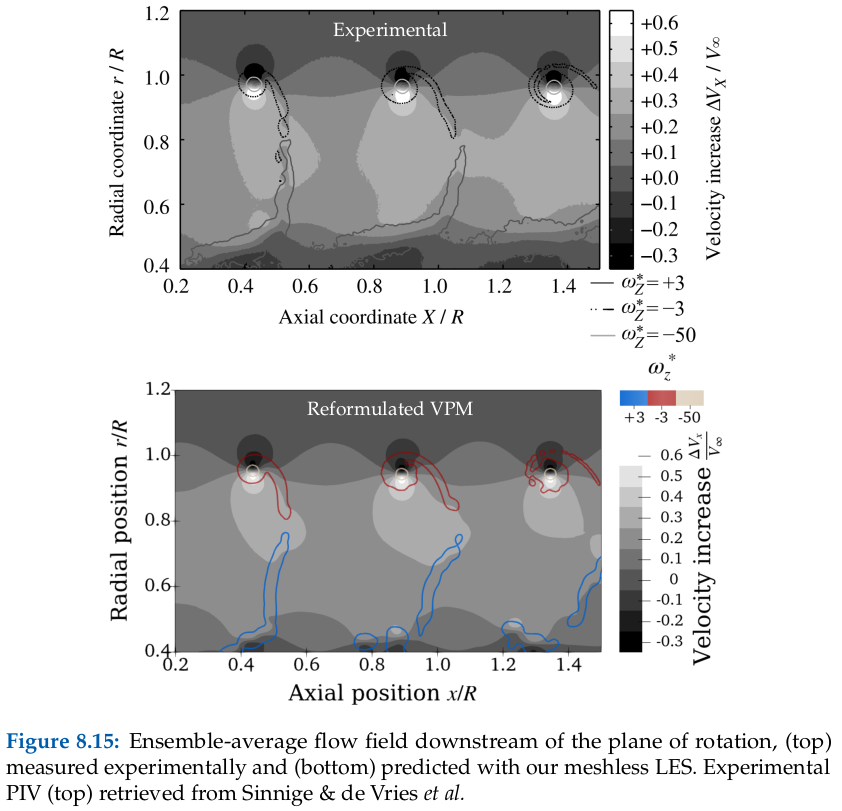
Flow field
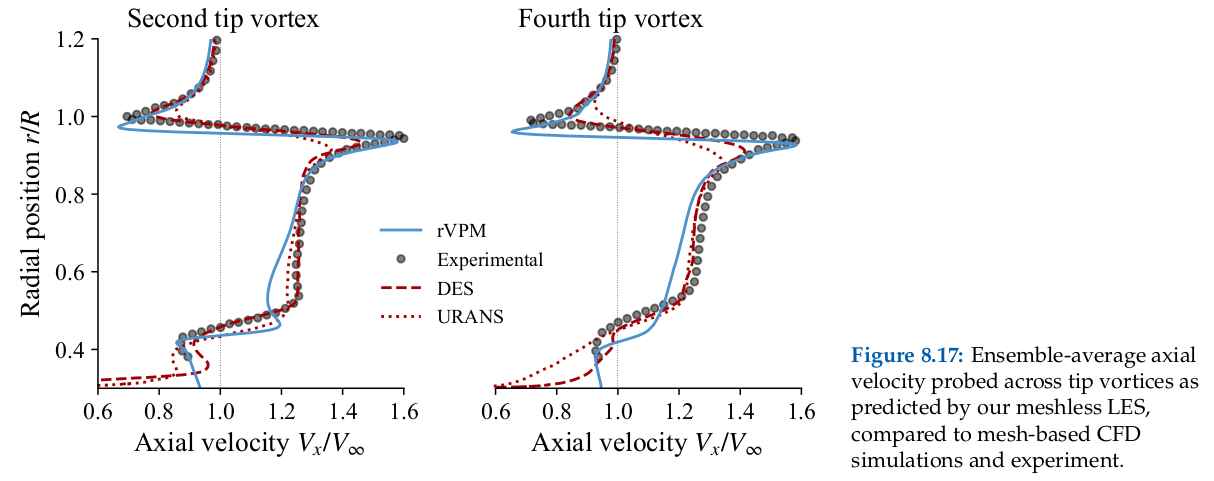
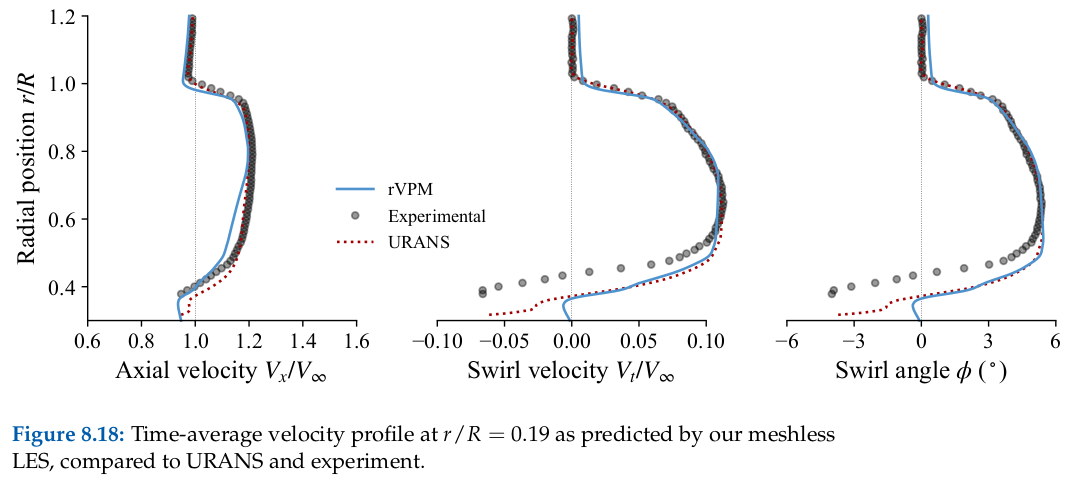
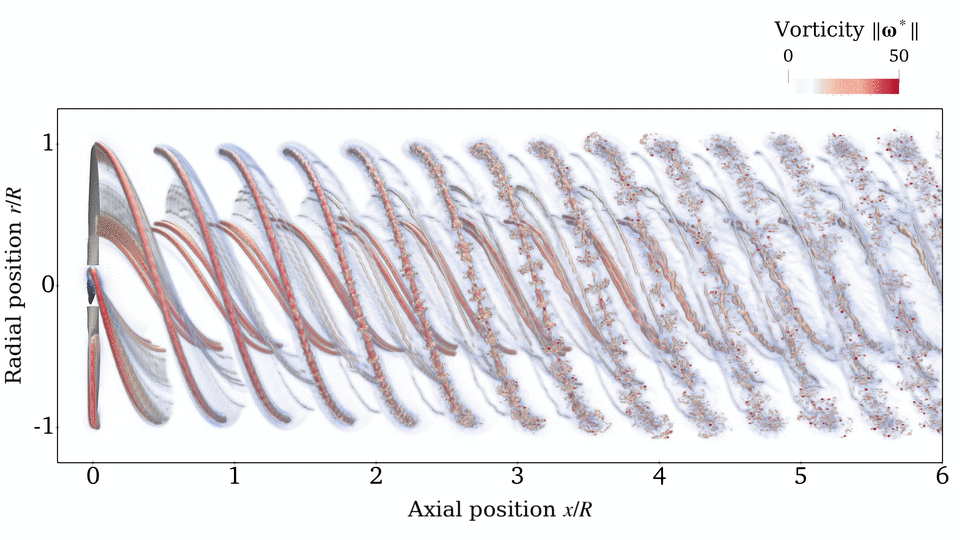
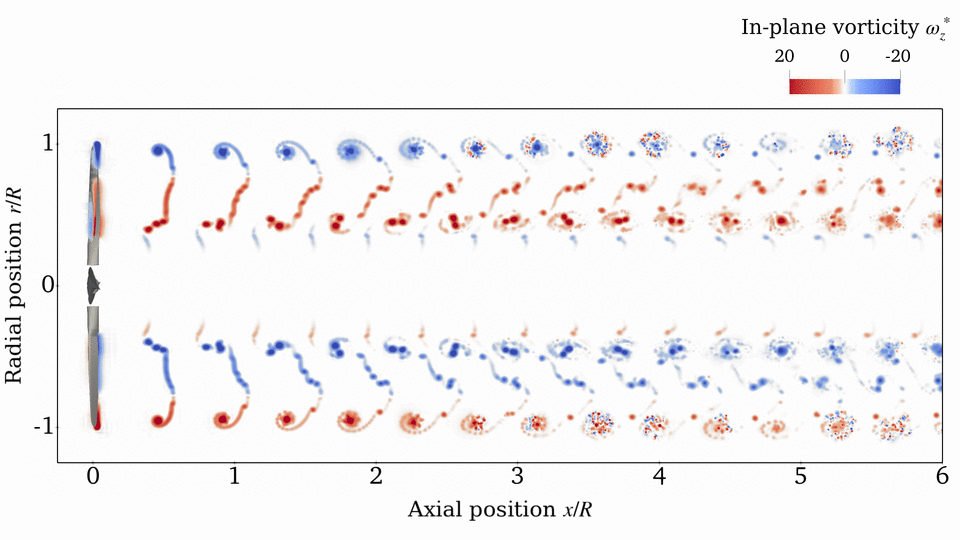
Rotor-Rotor Interactions
Side-by-Side Rotors in Hover
Source: E. J. Alvarez and A. Ning, 2020[3]
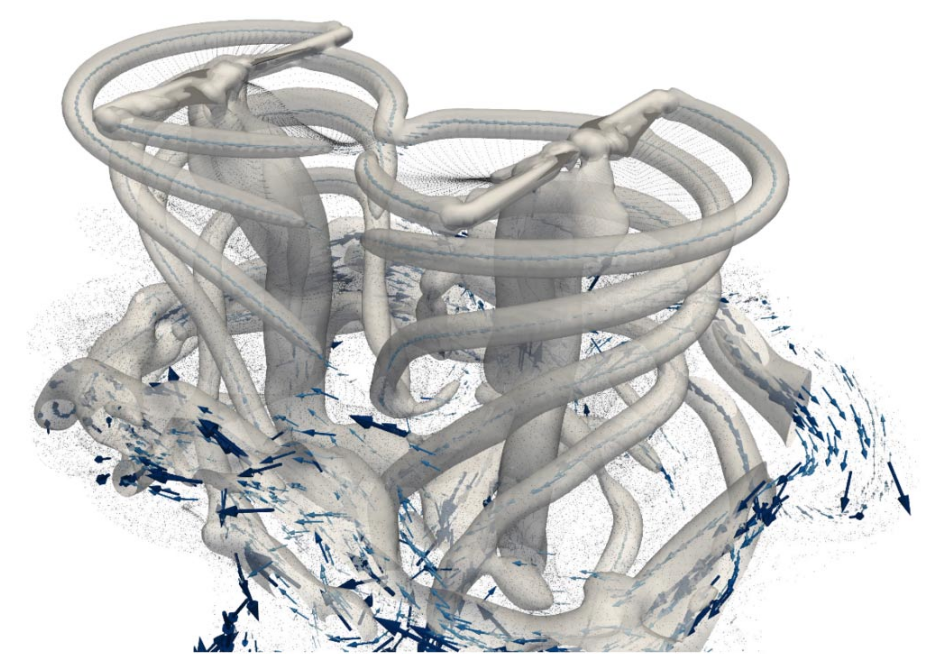
Case: Two side-by-side DJI rotors in hover. Two-bladed rotors with a diameter of 9.4 inches. Test at 4860 RPM, tip Mach number of 0.18, $\mathrm{Re}_c = 6.2\times 10^4$, and $\mathrm{Re}_{0.7D} = 6.5\times 10^5$. Aerodynamic interactions as the tip-to-tip spacing $s$ between rotors is decreased.
Results: FLOWUnsteady accurately captures both the drop in thrust and the increase in fluctuations as rotors are brought closer together.
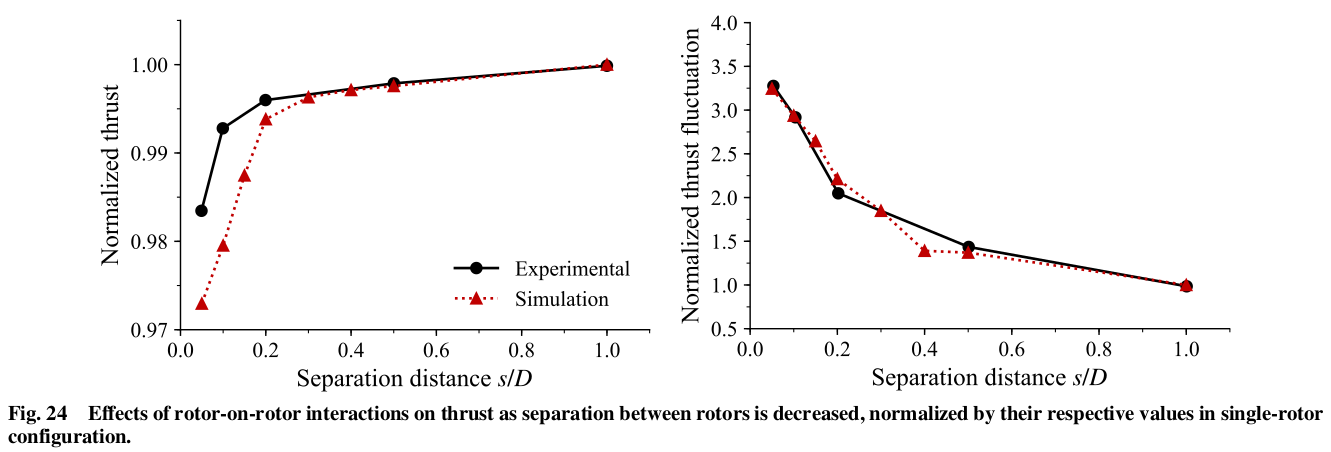
Side-by-Side and Tandem Propeller
Source: R. M. Erhard and J. J. Alonso, 2022[8]
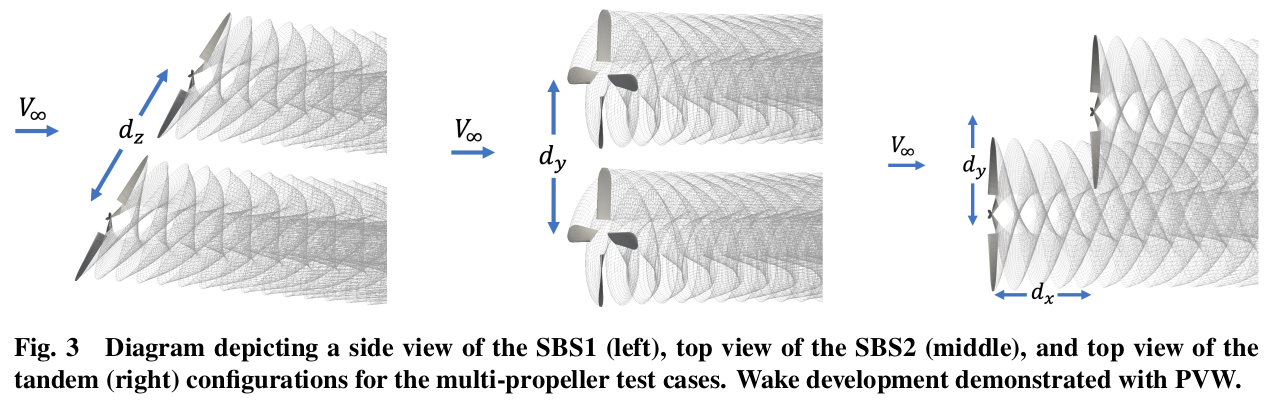
Case: Two TUD F29 propellers in side-by-side and tandem configurations at an multile incidence angles (edgewise flow). This is a four-bladed rotor. Thrust and power predicted by FLOWUnsteady (VPM) was compared to experiment and low-fidelity methods at multiple incidence angles $\alpha$ and advance ratios $J$.
Results: Good agreement between FLOWUnsteady and experiment for incidence angles up to $\alpha=60^\circ$ across advance ratio.
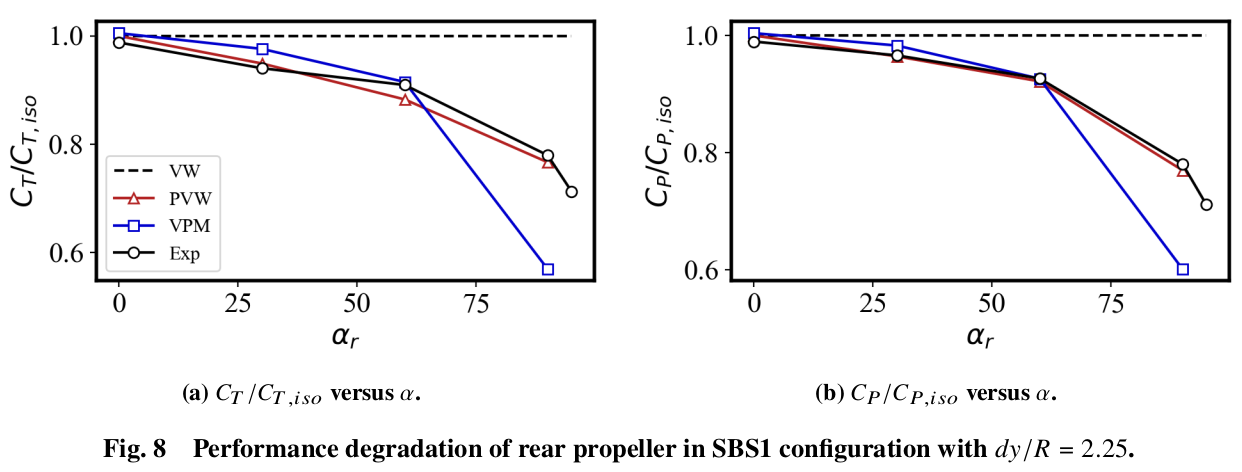
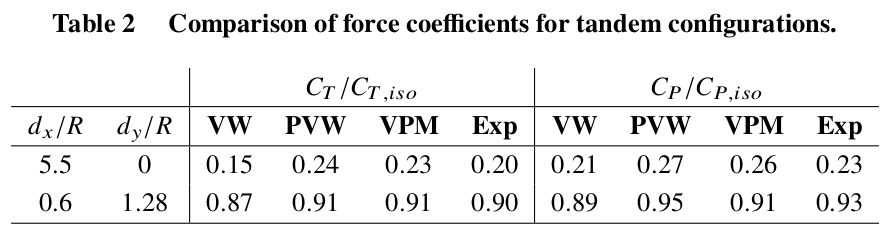
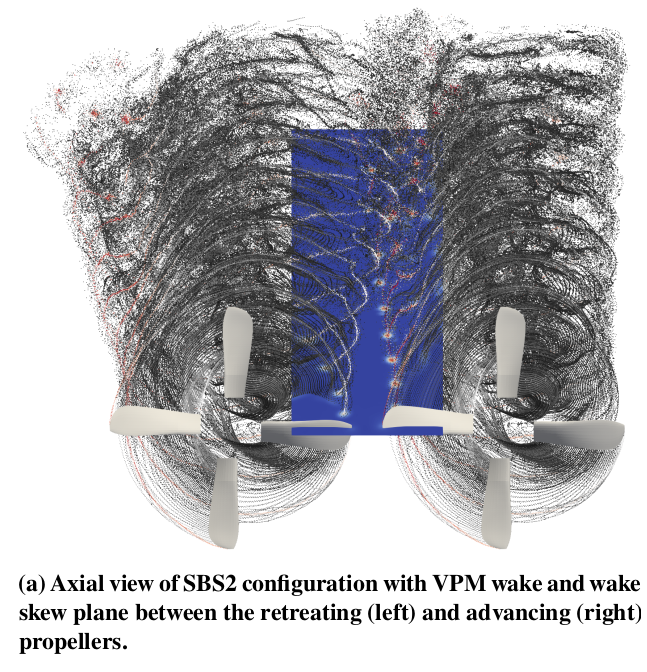
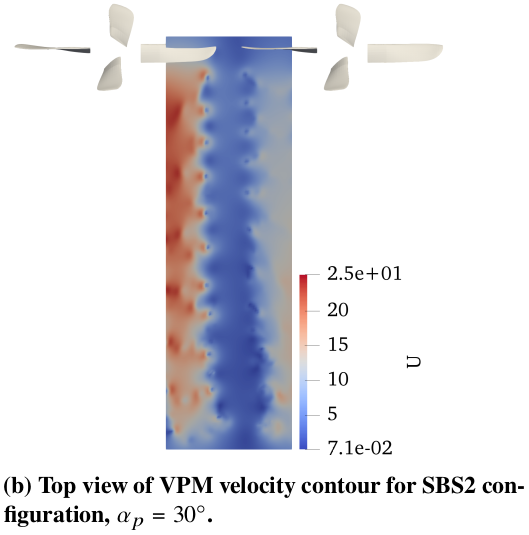
Rotor-Wing Interactions
Tailplane w/ Tip-Mounted Propellers
Source: E. J. Alvarez, 2022[1], and E. J. Alvarez and A. Ning, 2023[2]
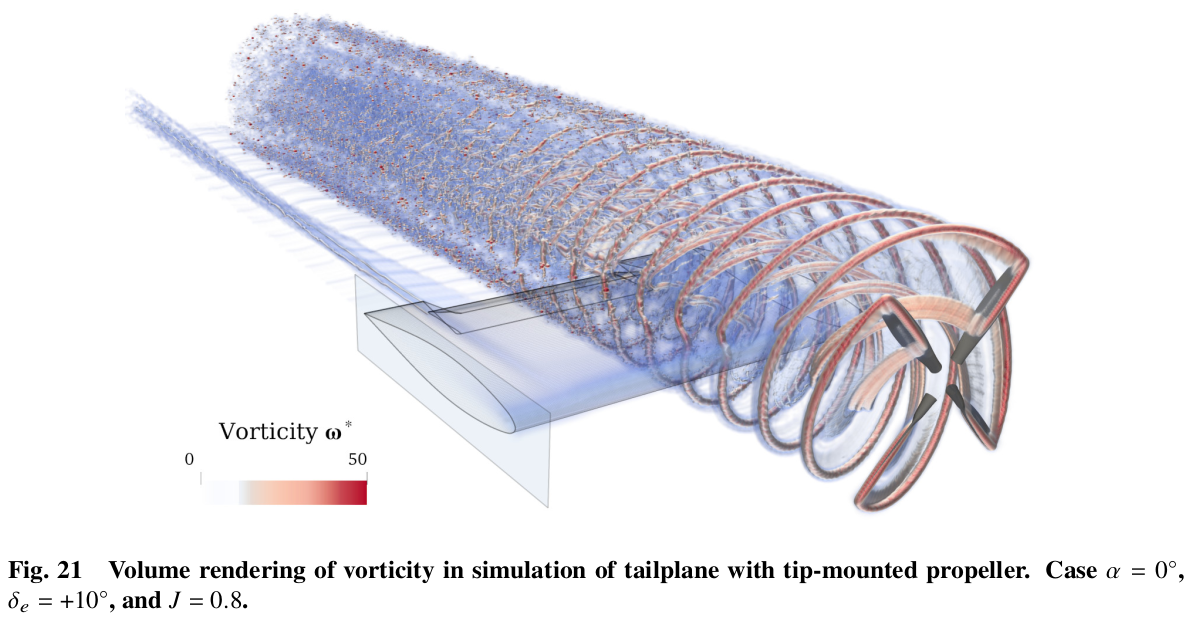
Case: Horizontal stabilizer (tailplane) with tip-mounted Beaver propellers. Wing with low aspect ratio ($b/c=2.7$), symmetric NACA $64_2-\mathrm{A}015$ profile, and elevator flap. Test at advance ratio $J$ of 0.8 and inboard-up propeller rotation at a thrust setting of $C_T = 0.0935$. Flap deflection captured in the actuator surface model (ASM) through an equivalent twist. Effects of wake impingement on wing loading predicted by FLOWUnsteady are compared to experiment.
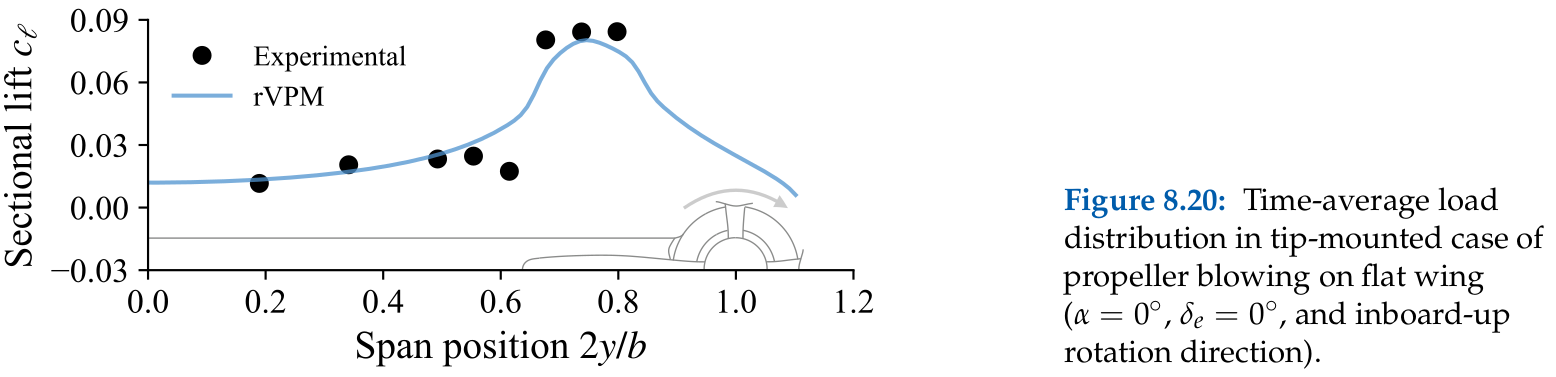
Lift augmentation
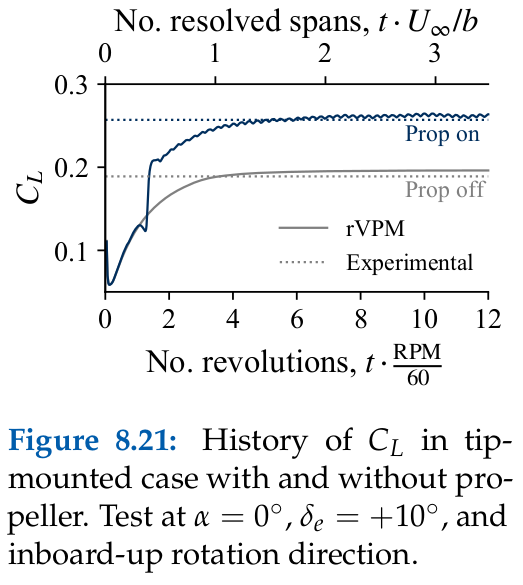
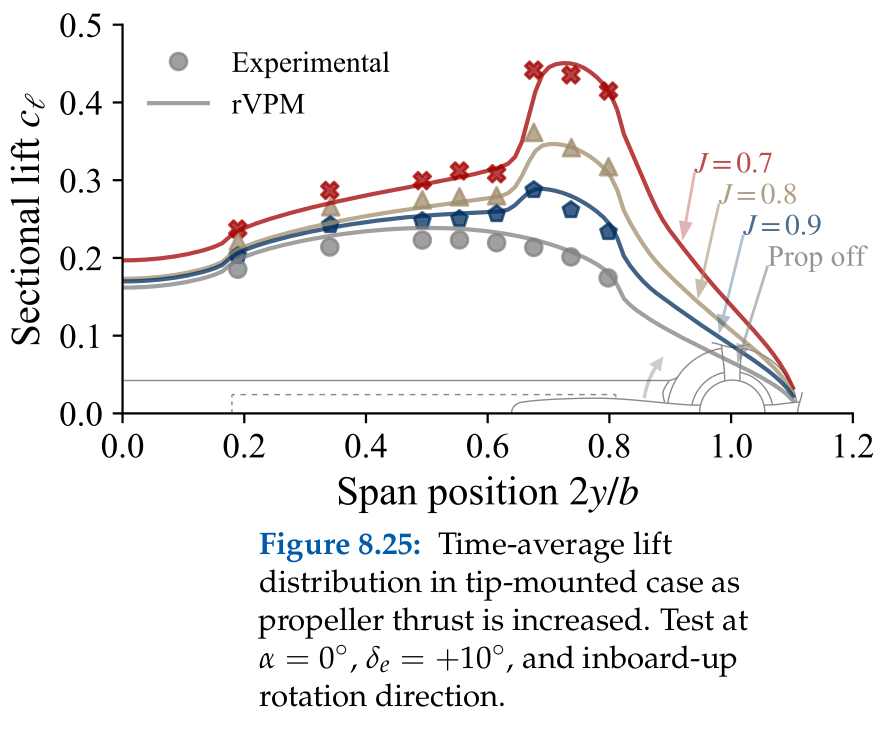
Wing wake and Streamtube
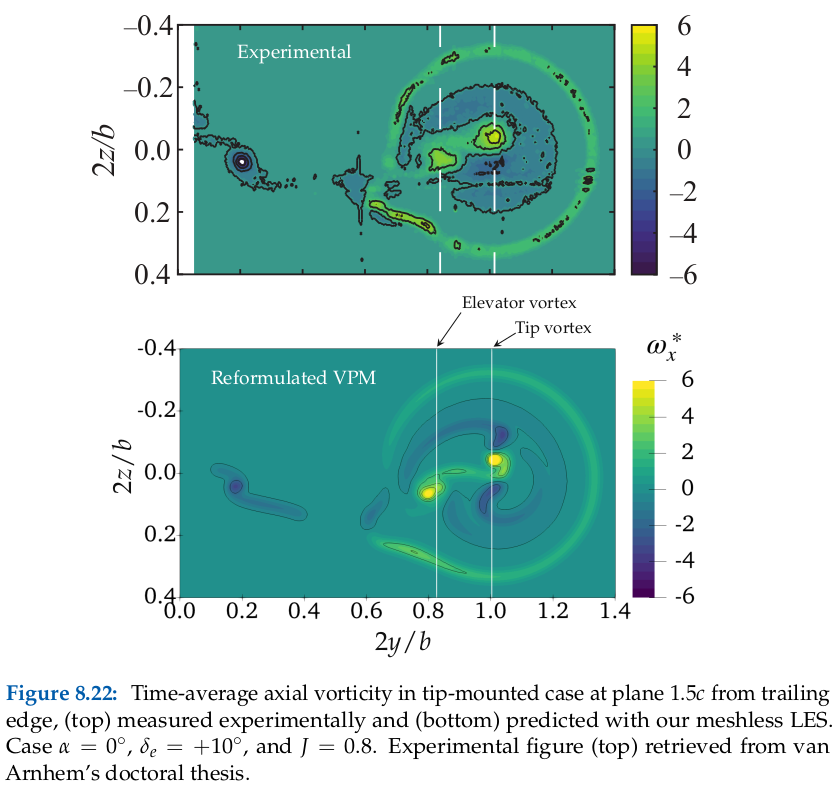
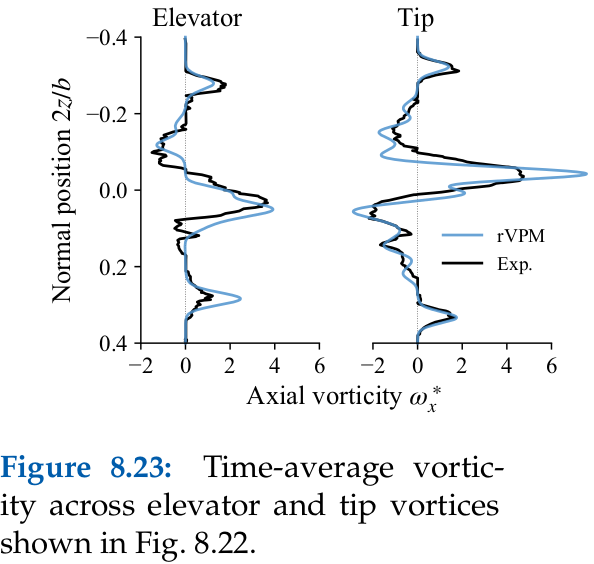
Effects of swirl direction
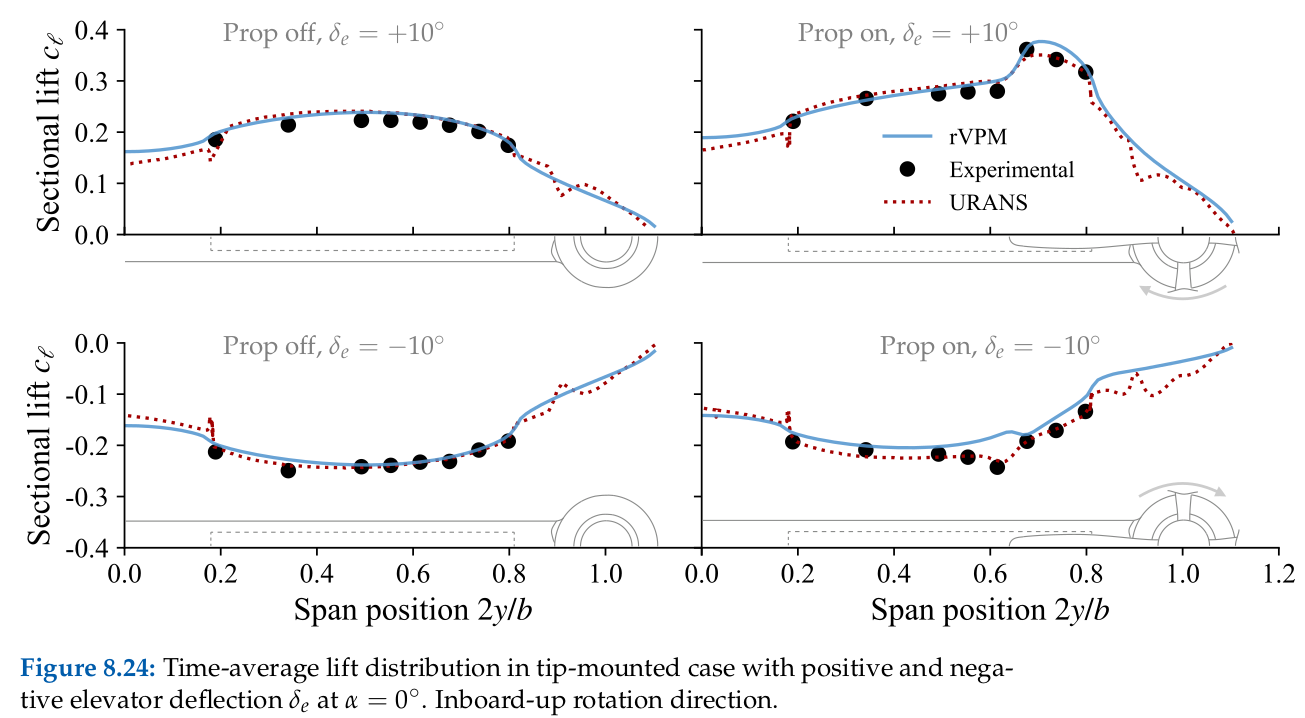
Strong impingement
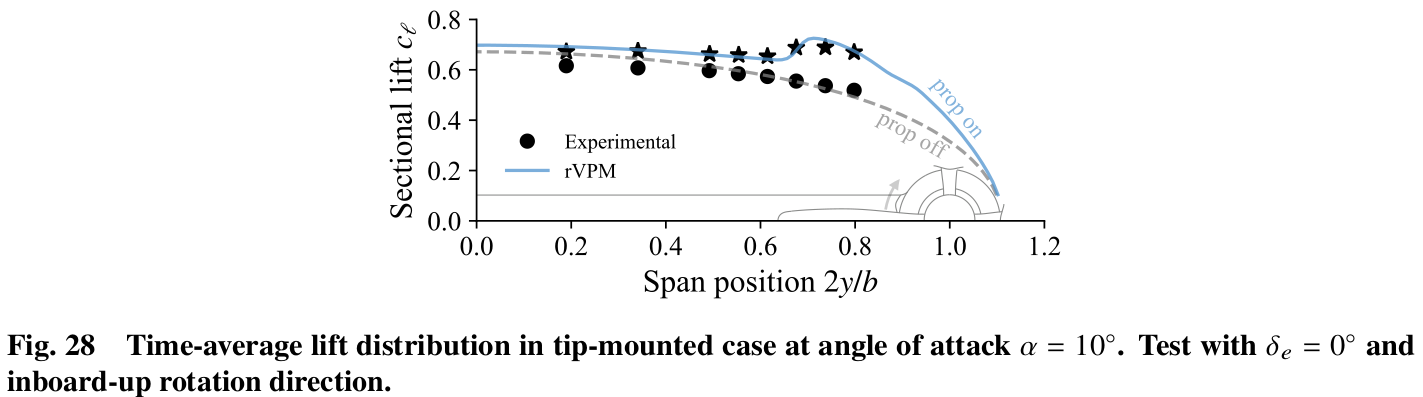
Blown Wing
Source: E. J. Alvarez, 2022[1], and E. J. Alvarez and A. Ning, 2023[2]
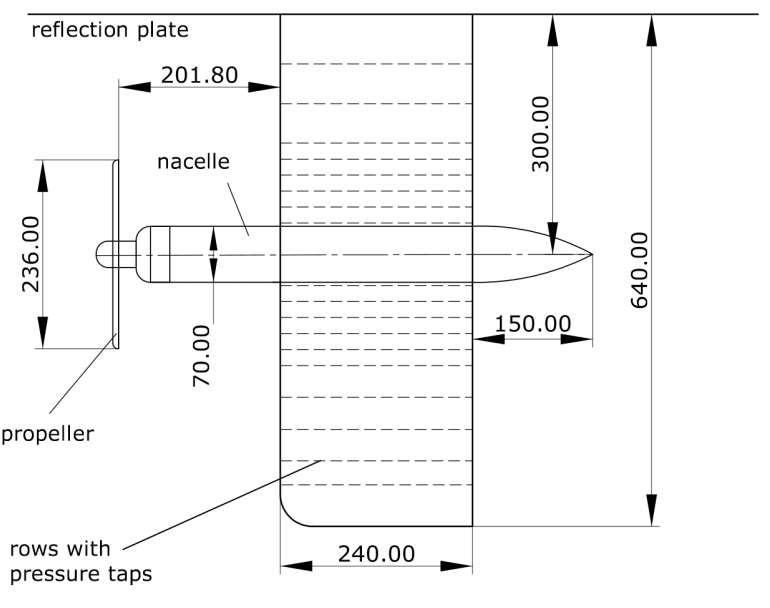
Case: Straight wing with Beaver propellers mounted mid-span. Wing of aspect ratio $b/c=5.33$, symmetric NACA $64_2-\mathrm{A}015$ profile. Test at advance ratio $J$ of 0.85 and inboard-up propeller rotation at a thrust setting of $C_T = 0.121$. Effects of wake impingement on wing loading predicted by FLOWUnsteady are compared to experiment.
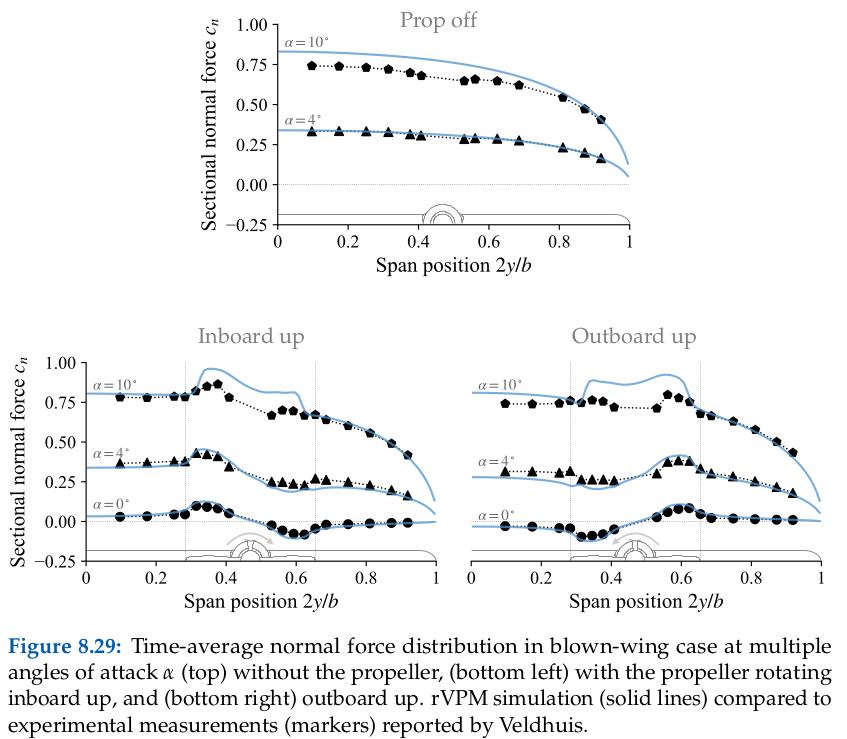
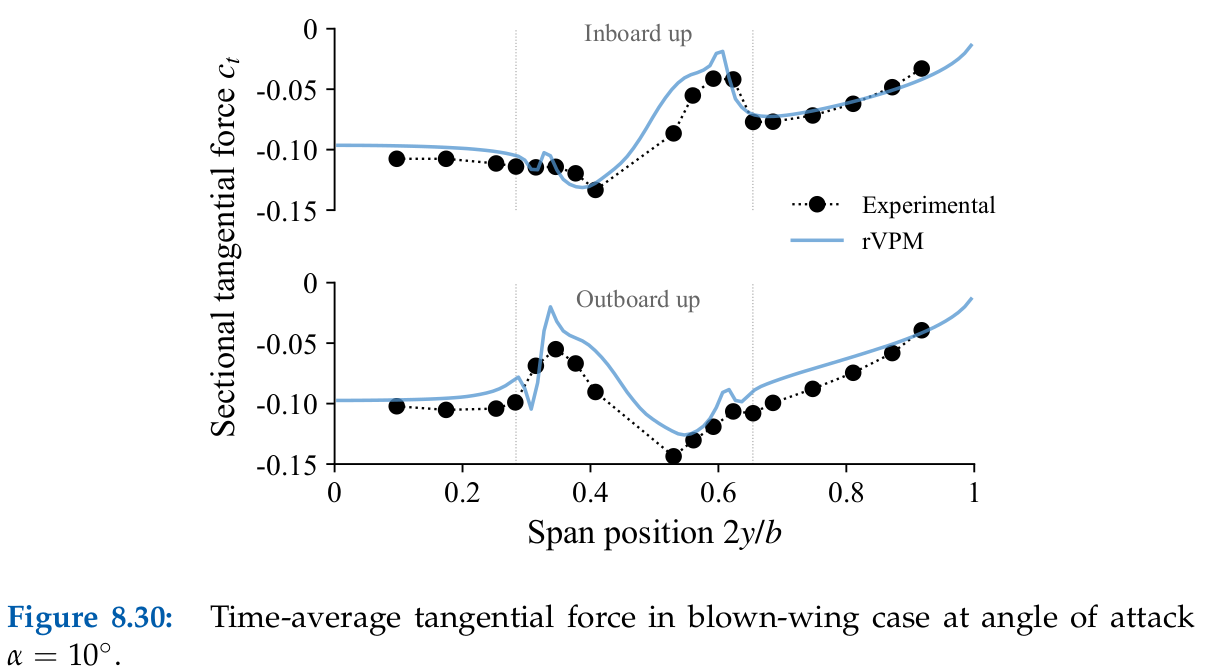
- 1E. J. Alvarez (2022), "Reformulated Vortex Particle Method and Meshless Large Eddy Simulation of Multirotor Aircraft," Doctoral Dissertation, Brigham Young University. [PDF]
- 2E. J. Alvarez & A. Ning (2023), "Meshless Large Eddy Simulation of Propeller-Wing Interactions Through the Reformulated Vortex Particle Method," Journal of Aircraft. [DOI] [PDF]
- 3E. J. Alvarez & A. Ning (2020), "High-Fidelity Modeling of Multirotor Aerodynamic Interactions for Aircraft Design," AIAA Journal. [DOI] [PDF]
- 5J. Mehr, E. J. Alvarez, & A. Ning (2022), "Interactional Aerodynamics Analysis of a Multi-Rotor Energy Kite," (in review).
- 6E. J. Alvarez & A. Ning (2023), "Stable Vortex Particle Method Formulation for Meshless Large-Eddy Simulation," AIAA Journal. [DOI] [PDF]
- 7E. J. Alvarez, A. Schenk, T. Critchfield, and A. Ning (2020), “Rotor-on-Rotor Aeroacoustic Interactions of Multirotor in Hover,” VFS 76th Forum. [PDF]
- 8R. M. Erhard and J. J. Alonso (2022), "Comparison of Propeller Wake Models for Distributed Electric Propulsion and eVTOL Aircraft in Complex Flow Conditions," AIAA SciTech Forum. [PDF]